There is such a compound: tartaric acid. It is a waste of the wine industry. Initially, tartaric acid is contained in grape juice in the form of its acidic sodium salt. However, during the fermentation process, sugar under the influence of special yeast turns into alcohol, and from this the solubility of the tartaric acid salt decreases. Then it precipitates, which is called tartar. It crystallizes, acidifies and, in the end, the acid itself is obtained. However, it is not so simple.
Pasteur
In fact, the solution contains two acids: tartaric and the other, grape. They differ in that tartaric acid has optical activity (it rotates the plane of polarized light to the right), while grape acid does not. Louis Pasteur investigated this phenomenon and found that the crystals formed by each of the acids are mirror images of each other, that is, he suggested a connection between the shape of the crystals and the optical activity of substances. In 1848, after a series of experiments, he announced a new type of tartaric acid isomerism, which he called enantiomerism.
Van goff
Jacob Vant-Hoff introduced the concept of the so-called asymmetric (or chiral) carbon atom. This is such carbon, which in an organic molecule is bound to four different atoms. For example, in tartaric acid, the second atom in the chain has a carboxyl group, hydrogen, oxygen and a second piece of tartaric acid in its neighbors. Since carbon has its bonds in the form of a tetrahedron in this configuration, it is possible to obtain two compounds that will be mirror images of each other, but it will be impossible to “superimpose” them on one another without changing the order of bonds in the molecule. By the way, such a way to determine chirality is Lord Kelvin’s suggestion: displaying a group of points (in our case, points are atoms in a molecule) possessing chirality in an ideal flat mirror cannot be combined with the group of points itself.
Molecular symmetry
The explanation with the mirror looks simple and beautiful, but in modern organic chemistry, where really huge molecules are studied, this speculative method is associated with significant difficulties. Therefore, they turn to mathematics. More precisely, to symmetry. There are so-called symmetry elements - axis, plane. We twist and rotate the molecule, leaving the symmetry element motionless, and the molecule after rotation through a certain angle (360 °, 180 ° or something else) begins to look exactly the same as at the beginning.
And the very asymmetric carbon atom introduced by Vant-Hoff is the basis of the simplest form of symmetry. This atom is the chiral center of the molecule. It is tetrahedral: it has four bonds with different substituents on each. And therefore, turning the compound along the axis containing such an atom, we will get an identical picture only after a complete rotation of 360 °.
In general, not only one atom can be the chiral center of a molecule. For example, there is such an interesting compound - adamantane. It looks like a tetrahedron, in which each edge is additionally arched outward, and in each corner there is a carbon atom. The tetrahedron is symmetrical about its center, and the adamantane molecule is also. And if four different substituents are added to the four identical “nodes” of adamantane, then he will also find point symmetry. Indeed, if you rotate it relative to its internal "center of gravity", the picture will coincide with the initial one only through 360 °. Here, instead of the asymmetric atom, the role of the chiral center is played by the “empty” center of adamantane.
Stereoisomers in Bioorganic Compounds
Chirality is an extremely important property for biologically active compounds. In the processes of life only isomers with a certain structure are involved. And almost all substances significant for the body are arranged in such a way that they have at least one chiral center. The most popular example is sugar. Here is the glucose. Its chain has six carbon atoms. Of these, four atoms have four different substituents nearby. Therefore, there are 16 possible optical isomers for glucose in total. All of them are divided into two large groups according to the configuration of the asymmetric carbon atom closest to the alcohol group: D-saccharides and L-saccharides. Only D-saccharides are involved in metabolic processes in a living organism.
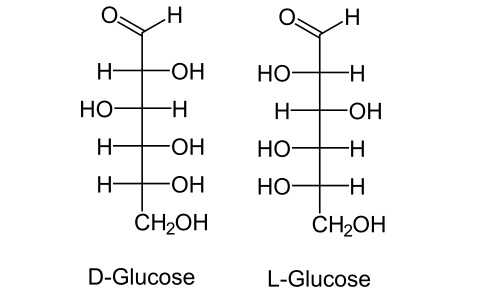
Also an example quite common for stereoisomerism in bioorganic chemistry is amino acids. All natural amino acids have amino groups near the carbon atom closest to the carboxyl group. Thus, in any amino acid, this atom will be asymmetric (various substituents are a carboxyl group, an amino group, hydrogen and the rest of the chain; the exception is glycine with two hydrogen atoms).
Accordingly, according to the configuration of this atom, all amino acids are also divided into D-series and L-series, only in natural processes, unlike sugars, the L-series prevails.