The spectrum of synchrotron radiation is not that great. That is, it can only be divided into several types. If the particle is nonrelativistic, then such radiation is called cyclotron emission. If, on the other hand, particles are inherently relativistic, then the radiation resulting from their interaction is sometimes called ultrarelativistic. Synchronous radiation can be achieved either artificially (in synchrotrons or storage rings), or naturally due to fast electrons moving through magnetic fields. The radiation thus obtained has a characteristic polarization, and the generated frequencies can vary across the entire electromagnetic spectrum, which is also called continuum radiation.
Opening
This phenomenon was named after the General Electric synchrotron generator, built in 1946. Its existence was announced in May 1947 by scientists Frank Elder, Anatoly Gurevich, Robert Langmuir and Coat of Arms Pollock in a letter "Electron Radiation in the Synchrotron." But this was only a theoretical discovery; you will read about the first real observation of this phenomenon below.
Sources
When particles with high energy are in acceleration, including electrons, forced to move along a curved path by a magnetic field, synchrotron radiation is obtained. This is similar to the radio antenna, but with the difference that theoretically the relativistic velocity will change the observed frequency due to the Doppler effect on the Lorentz coefficient γ. The reduction in the relativistic length then hits the frequency observed by another factor γ, thereby increasing the frequency of the GHz resonant cavity, which accelerates the electrons in the x-ray range. The radiated power is determined by the relativistic Larmor formula, and the force on the radiated electron is determined by the force of Abraham-Lorentz-Dirac.
Other characteristics
The radiation pattern can be distorted from an isotropic dipole pattern into an extremely directional radiation cone. Synchrotron electron radiation is the brightest artificial source of x-rays.
The geometry of planar acceleration, apparently, makes the radiation linearly polarized when observed in the plane of the orbit and circularly polarized when observed at a small angle to this plane. Amplitude and frequency, however, are concentrated on the polar ecliptic.
A source of synchrotron radiation is also a source of electromagnetic radiation (EM), which is a storage ring created for scientific and technical purposes. This radiation is produced not only by storage rings, but also by other specialized particle accelerators, usually accelerating electrons. As soon as a high-energy electron beam is generated, it is aimed at auxiliary components, such as bending magnets and insertion devices (undulators or wigglers). They provide strong magnetic fields, perpendicular rays, which are necessary for the conversion of high-energy electrons into photons.
The use of synchrotron radiation
The main fields of application of synchrotron light are condensed matter physics, materials science, biology and medicine. Most of the experiments using synchrotron light involve studying the structure of a substance from the sub-nanometer level of the electronic structure to the level of a micrometer and millimeter, which is important for medical imaging. An example of practical industrial application is the production of microstructures according to the LIGA process.
Synchrotron radiation is also generated by astronomical objects, usually where relativistic electrons spiral (and therefore change speed) through magnetic fields.
History
This radiation was first discovered in a missile launched by Messier 87 in 1956, Jeffrey R. Bourges, who saw it as confirmation of the prediction of Joseph Shklovsky in 1953, but it was previously predicted by Hannes Alfven and Nikolai Herlofson in 1950. Solar flares accelerate particles that emit in the manner proposed by R. Giovanolli in 1948 and critically described by Piddington in 1952.
Space
Supermassive black holes are proposed for creating synchrotron radiation by pushing jets created by gravitationally accelerated ions through super-sorted “tubular” polar regions of magnetic fields. Such jets, coming from them in Messier 87, were determined by the Hubble telescope as superluminal signals moving at a frequency of 6 × s (six times the speed of light) from our planetary frame. This phenomenon is caused by the fact that the jets move very close to the speed of light and at a very small angle to the observer. Since high-speed jets emit light at each point of their path, the light that they emit does not approach the observer much faster than the jet itself. The light emitted during hundreds of years of travel thus comes to the observer over a much shorter period of time (ten or twenty years). There is no violation of the special theory of relativity in this phenomenon.
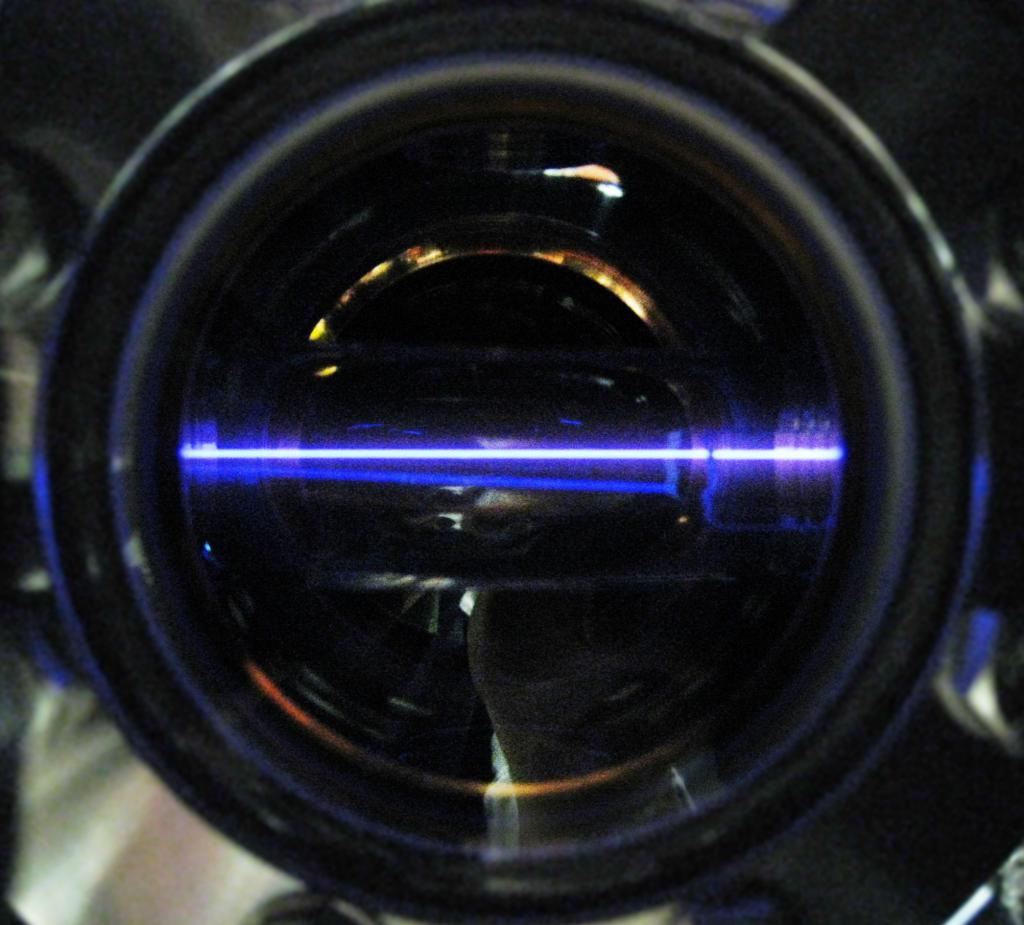
Recently, pulsed emission of gamma radiation from a nebula with a brightness of up to ≥25 GeV has been discovered, probably due to synchrotron radiation by electrons captured by a strong magnetic field around the pulsar. The class of astronomical sources where synchrotron emission is important is pulsar wind nebulae, or plerions, of which the Crab nebula and the associated pulsar are archetypal. Polarization in the Crab nebula at energies from 0.1 to 1.0 MeV is typical synchrotron radiation.
Briefly about calculation and colliders
In equations on this subject, special terms or values are often written that symbolize the particles that make up the so-called velocity field. These terms represent the effect of a static field of a particle, which is a function of a component of its motion, which has zero or constant velocity. On the contrary, the second term falls as the inverse first power of the distance from the source, and some terms are called the acceleration field or the radiation field, because they are components of the field arising from the acceleration of the charge (change in speed).
Thus, the radiated power is scaled as fourth-degree energy. This radiation limits the energy of the electron-positron circular collider. Typically, proton colliders are instead limited by the maximum magnetic field. Therefore, for example, the Large Hadron Collider has a center of mass energy 70 times higher than any other particle accelerator, even if the proton mass is 2,000 times the electron mass.
Terminology
Different fields of science often have different ways of defining terms. Unfortunately, in the field of x-rays, several terms mean the same thing as "radiation". Some authors use the term “brightness”, which was once used to indicate photometric brightness or was used incorrectly to mean radiometric radiation. Intensity means power density per unit area, but for x-ray sources it usually means brilliance.
Mechanism of occurrence
Synchrotron radiation can occur in accelerators either as an unforeseen error, causing undesirable energy losses in the context of particle physics, or as a deliberately created radiation source for numerous laboratory applications. Electrons are accelerated to high speeds in several stages to achieve the final energy, which is usually in the giga-electronvolt range. Electrons are forced to move in a closed path by strong magnetic fields. This is similar to the radio antenna, but with the difference that the relativistic velocity changes the observed frequency due to the Doppler effect. Relativistic Lorentz compression affects the gigahertz frequency, thereby multiplying it in the resonant cavity, which accelerates the electrons in the x-ray range. Another dramatic effect of relativity is that the radiation pattern is distorted from the isotropic dipole pattern expected from a nonrelativistic theory to an extremely directional radiation cone. This makes synchrotron radiation diffraction the best way to create x-rays. The plane acceleration geometry makes the radiation linearly polarized when observed in the plane of the orbit and creates circular polarization when observed at a small angle to this plane.
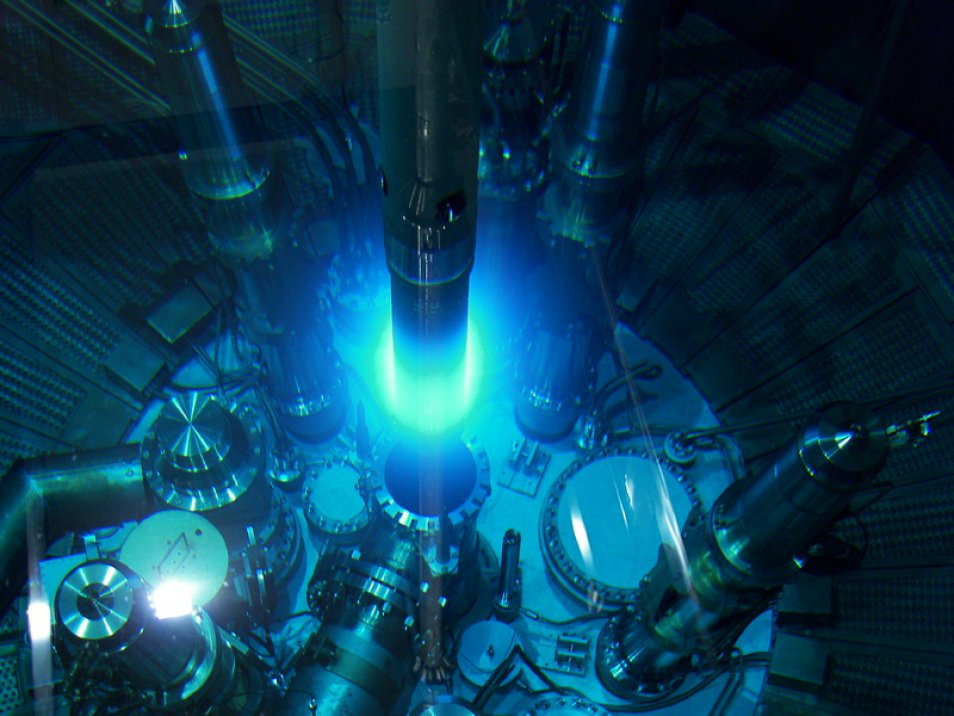
Use in various fields
The benefits of using synchrotron radiation for spectroscopy and diffraction have been realized by the ever-growing scientific community since the 1960s and 1970s. Initially, particle accelerators were created for particle physics. In the "parasitic mode" synchrotron radiation was used, when the bending magnetic radiation had to be extracted by drilling additional holes in the beam tubes. The first storage ring introduced as a synchrotron light source was Tantalus, which was first launched in 1968. As accelerator radiation became more intense and its applications became more promising, devices that enhanced its intensity were built into existing rings. The synchrotron radiation diffraction method was developed and optimized from the very beginning to obtain high-quality x-rays. The sources of the fourth generation are considered, which will include various concepts for creating ultra-diamond, pulsed temporal structural X-rays for extremely demanding and, possibly, not yet created experiments.
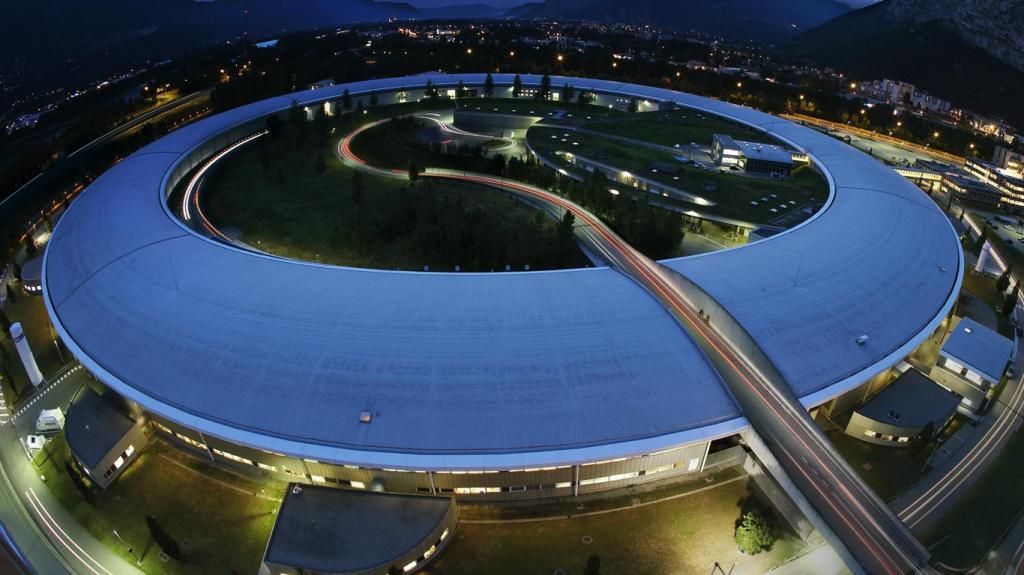
First devices
At first, bending electromagnets were used in accelerators to generate this radiation, but sometimes other specialized devices — insertion devices — were sometimes used to create a stronger light effect. Diffraction methods of synchrotron radiation (third generation) usually depend on source devices, where the straight sections of the storage ring contain periodic magnetic structures (containing many magnets in the form of alternating poles N and S) that cause the electrons to move in a sinusoidal or spiral way. Thus, instead of a single bend, many tens or hundreds of “twists” in precisely calculated positions add up or multiply the total beam intensity. These devices are called wigglers or undulators. The main difference between the undulator and wiggler is the intensity of their magnetic field and the amplitude of the deviation from the direct path of the electrons. All these devices and mechanisms are now stored in the Synchrotron Radiation Center (USA).
Extraction
There are holes in the storage ring that allow particles to escape from the radiation background and follow the line of the beam into the experimenter's vacuum chamber. A large number of such rays can appear from modern third-generation synchrotron radiation devices.
Electrons can be extracted from the accelerator itself and stored in an auxiliary magnetic storage device of ultra-high vacuum, from where they can be extracted (and where they can be reproduced) a large number of times. The magnets in the ring must also repeatedly compress the beam against the "Coulomb forces" (or, more simply, space charges), which tend to destroy electron bunches. A change in direction is a form of acceleration, because electrons produce radiation at high energies and a high acceleration rate in a particle accelerator. As a rule, the brightness of synchrotron radiation also depends on the same speed.