In the study and practical use of atomic phenomena, one of the most important roles is played by x-rays. Thanks to their research, many discoveries were made and methods for the analysis of substances used in various fields were developed. Here we look at one of the types of x-rays - characteristic x-rays.
The nature and properties of x-rays
X-ray radiation is a high-frequency change in the state of an electromagnetic field propagating in space at a speed of about 300,000 km / s, that is, electromagnetic waves. On the scale of the range of electromagnetic radiation, the x-ray is located in the wavelength region from about 10 -8 to 5 β 10 -12 meters, which is several orders of magnitude shorter than optical waves. This corresponds to frequencies from 3 β 10 16 to 6 β 10 19 Hz and energies from 10 eV to 250 keV, or 1.6 β 10 -18 to 4 β 10 -14 J. It should be noted that the boundaries of the frequency ranges of electromagnetic radiation are rather arbitrary due to their overlap.
The source of x-ray radiation is the interaction of accelerated charged particles (high-energy electrons) with electric and magnetic fields and with atoms of matter.
X-ray photons are characterized by high energies and a large penetrating and ionizing ability, especially for hard X-rays with wavelengths less than 1 nanometer (10 -9 m).
X-rays interact with matter, ionizing its atoms, in the processes of the photoelectric effect (photoabsorption) and incoherent (Compton) scattering. During photoabsorption, an x-ray photon, absorbed by the electron of the atom, transfers energy to it. If its value exceeds the binding energy of an electron in an atom, then it leaves the atom. Compton scattering is characteristic of harder (energetic) X-ray photons. Part of the energy of the absorbed photon is spent on ionization; in this case, at a certain angle to the direction of the primary photon, a secondary one is emitted, with a lower frequency.
Types of x-ray radiation. Brake light
To obtain rays using x-ray tubes, which are glass vacuum cylinders with electrodes located inside. The potential difference at the electrodes is needed very high - up to hundreds of kilovolts. Thermoelectronic emission occurs at the tungsten cathode heated by current, that is, electrons are emitted from it, which, accelerated by the potential difference, bombard the anode. As a result of their interaction with the atoms of the anode (sometimes called the anticathode), X-ray photons are born.
Depending on what process leads to the birth of a photon, there are distinguished types of x-ray radiation, such as bremsstrahlung and characteristic.
When electrons encounter the anode, they can slow down, that is, lose energy in the electric fields of its atoms. This energy is emitted in the form of x-ray photons. Such radiation is called bremsstrahlung.
It is clear that the braking conditions will vary for individual electrons. This means that different amounts of their kinetic energy are converted to x-ray radiation. As a result, bremsstrahlung includes photons of different frequencies and, accordingly, wavelengths. Therefore, its spectrum is continuous (continuous). Sometimes for this reason it is also called βwhiteβ x-ray radiation.
The energy of the bremsstrahlung photon cannot exceed the kinetic energy of the electron that generates it, so that the maximum frequency (and the smallest wavelength) of the bremsstrahlung corresponds to the highest kinetic energy of the electrons incident on the anode. The latter depends on the potential difference applied to the electrodes.
There is another type of x-ray, the source of which is a different process. This radiation is called characteristic, and we will dwell on it in more detail.
How does characteristic x-ray radiation arise
Having reached the anticathode, a fast electron can penetrate inside the atom and knock out an electron from one of the lower orbitals, that is, transfer to it enough energy to overcome the potential barrier. However, if there are higher energy levels in the atom occupied by electrons, the vacated space will not remain empty.
It must be remembered that the electronic structure of an atom, like any energy system, seeks to minimize energy. The vacancy formed as a result of knocking out is filled with an electron from one of the overlying levels. Its energy is higher, and, occupying a lower level, it emits an excess in the form of a quantum of characteristic x-ray radiation.
The electronic structure of an atom is a discrete set of possible energy states of electrons. Therefore, X-ray photons emitted during the replacement of electronic vacancies can also have only strictly defined energy values ββthat reflect the difference in levels. As a result of this, the characteristic x-ray radiation has a spectrum not of a continuous, but of a linear form. Such a spectrum allows us to characterize the substance of the anode - hence the name of these rays. It is due to spectral differences that it is clear what is meant by bremsstrahlung and characteristic x-rays.
Sometimes the excess energy is not radiated by the atom, but is spent on knocking out the third electron. This process - the so-called Auger effect - is more likely to occur when the electron binding energy does not exceed 1 keV. The energy of a liberated Auger electron depends on the structure of the energy levels of the atom; therefore, the spectra of such electrons are also discrete.
General view of the characteristic spectrum
Narrow characteristic lines are present in the X-ray spectral pattern along with a solid stopping spectrum. If we present the spectrum in the form of a graph of the dependence of the intensity on the wavelength (frequency), in the locations of the lines we will see sharp peaks. Their position depends on the material of the anode. These maxima are present at any potential difference - if there are x-rays, there are always peaks too. With increasing voltage at the tube electrodes, the intensity of both continuous and characteristic x-ray radiation increases, but the location of the peaks and the ratio of their intensities does not change.
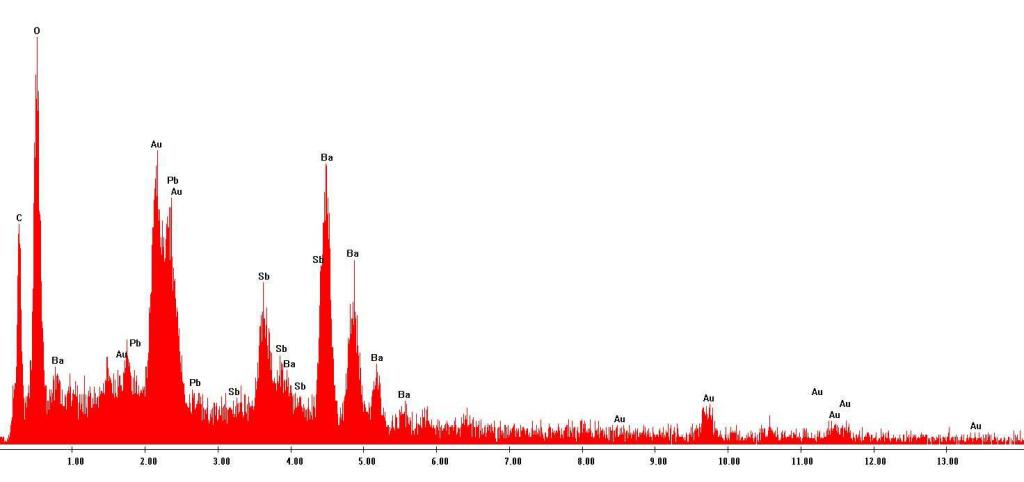
The peaks in the X-ray spectra have the same form, regardless of the material of the anticathode irradiated by electrons, but for different materials they are located at different frequencies, uniting in a series by the proximity of the frequency values. Between the series themselves, the difference in frequencies is much larger. The type of maxima does not depend on whether the anode material is a pure chemical element or is it a complex substance. In the latter case, the characteristic X-ray spectra of the constituent elements are simply superimposed on each other.
With an increase in the serial number of a chemical element, all lines of its X-ray spectrum shift towards an increase in frequency. The spectrum at the same time retains its appearance.
Mosley's Law
The phenomenon of the spectral shift of characteristic lines was experimentally discovered by the English physicist Henry Moseley in 1913. This allowed him to associate the frequencies of the spectrum maxima with the serial numbers of chemical elements. Thus, the wavelength of characteristic x-ray radiation, as it turned out, can be clearly correlated with a specific element. In general terms, the Moseley law can be written as follows: βf = (Z - S n ) / nβR, where f is the frequency, Z is the element number, S n is the screening constant, n is the main quantum number and R is the constant Rydberg. This dependence is linear and on the Mosley diagram looks like a series of straight lines for each value of n.
The values ββof n correspond to individual series of peaks of characteristic x-ray radiation. Moseley's law allows one to establish the serial number of a chemical element irradiated by hard electrons from the measured wavelengths (they are uniquely related to the frequencies) of the maxima of the X-ray spectrum.
The structure of the electron shells of chemical elements is identical. This is indicated by the monotonicity of the shear change in the characteristic spectrum of x-ray radiation. The frequency shift does not reflect structural, but energy differences between the electron shells, unique to each element.
The role of Mosley's law in atomic physics
There are slight deviations from the strict linear relationship expressed by Mosley's law. They are associated, firstly, with the peculiarities of the order in which electron shells are filled in some elements, and, secondly, with relativistic effects of the motion of electrons of heavy atoms. In addition, when the number of neutrons in the nucleus (the so-called isotopic shift) changes, the position of the lines can slightly change. This effect made it possible to study in detail the atomic structure.
Mosley's law is extremely significant. Its consistent application to the elements of the periodic table established the regularity of increasing the serial number, corresponding to each small shift of characteristic maxima. This helped clarify the question of the physical meaning of the serial number of elements. The value of Z is not just a number: it is the positive electric charge of the nucleus, which is the sum of the unit positive charges of the particles that make up its composition. The correct placement of elements in the table and the presence of empty positions in it (then they still existed) received strong confirmation. The validity of the periodic law was proved.
Moseley's law, in addition, became the basis on which a whole line of experimental research arose - x-ray spectrometry.
The structure of the electron shells of the atom
Let us briefly recall how the electronic structure of an atom is structured. It consists of shells, denoted by the letters K, L, M, N, O, P, Q or by numbers from 1 to 7. The electrons within the shell are characterized by the same main quantum number n, which determines the possible values ββof energy. In the outer shells, the electron energy is higher, and the ionization potential for external electrons is correspondingly lower.
A shell includes one or more sublevels: s, p, d, f, g, h, i. In each shell, the number of sublevels increases by one compared to the previous one. The number of electrons in each sublevel and in each shell cannot exceed a certain value. They are characterized, in addition to the main quantum number, by the same value of the orbital quantum number, which determines the shape of the electron cloud. Sublevels are indicated with the shell to which they belong, for example, 2s, 4d, and so on.
The sublevel contains atomic orbitals, which are set, in addition to the main and orbital ones, by another quantum number - the magnetic number, which determines the projection of the orbital momentum of the electron on the direction of the magnetic field. One orbital can have no more than two electrons, differing in the value of the fourth quantum number - spin.
Let us consider in more detail how characteristic x-ray radiation arises. Since the origin of this type of electromagnetic emission is associated with phenomena occurring inside the atom, it is most convenient to describe it precisely in the approximation of electronic configurations.
The mechanism for generating characteristic x-rays
So, the cause of this radiation is the formation of electronic vacancies in the inner shells due to the penetration of high-energy electrons deep inside the atom. The probability that a hard electron will interact increases with an increase in the density of electron clouds. Therefore, a collision within the densely packed inner shells, for example, the lowest K-shell, will be most likely. Here, the atom is ionized, and a vacancy forms in the 1s shell.
This vacancy is filled with an electron from the shell with higher energy, the excess of which is carried away by an X-ray photon. This electron can βfallβ from the second shell L, from the third M and so on. This is how the characteristic series is formed, in this example, the K-series. An indication of where the electron filling the vacancy comes from is given in the form of a Greek index when designating a series. "Alpha" means that it comes from the L-shell, "beta" - from the M-shell. Currently, there is a tendency to replace Greek alphabetic indices with Latin ones, used to designate shells.
The alpha line intensity in the series is always the highest - this means that the probability of filling a vacancy from an adjacent shell is the highest.
Now we can answer the question, what is the maximum quantum energy of characteristic x-ray radiation. It is determined by the difference in the energy values ββof the levels between which the transition of the electron takes place, according to the formula E = E n 2 - E n 1 , where E n 2 and E n 1 are the energies of the electronic states between which the transition occurred. The highest value of this parameter is given by transitions of the K series from the highest levels of atoms of heavy elements. But the intensity of these lines (peak height) is the smallest, since they are the least likely.
If, due to insufficient voltage at the electrodes, the hard electron cannot reach the K level, it forms a vacancy at the L level, and a less energetic L series with long wavelengths is formed. Subsequent series are born in a similar way.
In addition, when a vacancy is filled as a result of an electronic transition, a new vacancy arises in the overlying envelope. This creates the conditions for generating the next series. Electronic vacancies move higher from level to level, and the atom emits a cascade of characteristic spectral series, while remaining ionized.
Fine structure of characteristic spectra
The atomic X-ray spectra of characteristic X-ray radiation are characterized by a fine structure, which is expressed, like in the optical spectra, in line splitting.
The fine structure is due to the fact that the energy level - the electron shell - is a set of closely spaced components - subshells. To characterize the subshells, one more internal quantum number j was introduced, which reflects the interaction of the intrinsic and orbital magnetic moments of the electron.
Due to the influence of the spin-orbit interaction, the energy structure of the atom is complicated, and as a result, the characteristic x-ray emission has a spectrum that is characterized by split lines with very closely spaced elements.
Fine structure elements are usually denoted by additional digital indexes.
The characteristic x-ray radiation has a feature reflected only in the fine structure of the spectrum. The transition of the electron to the lower energy level does not occur from the lower subshell of the overlying level. Such an event has a negligible probability.
The use of x-rays in spectrometry
This radiation, due to its features described by the Mosley law, underlies various X-ray spectral methods of analysis of substances. When analyzing the x-ray spectrum, either crystal radiation diffraction (wave dispersion method) or energy-absorbed x-ray photon-sensitive detectors (energy dispersive method) are used. Most electron microscopes are equipped with various X-ray spectrometric attachments.
Particularly high accuracy is characterized by wave dispersive spectrometry. Using special filters, the most intense peaks in the spectrum are distinguished, so that it is possible to obtain almost monochromatic radiation with a precisely known frequency. The anode material is selected very carefully to ensure that a monochromatic beam of the desired frequency is obtained. Its diffraction on the crystal lattice of the substance under study allows us to study the lattice structure with great accuracy. This method is also used in the study of DNA and other complex molecules.
-. . - , . , -, , . . , , . β , -.
, . , .