In nuclear processes, as is known, three types of fundamental interactions manifest themselves: strong, weak, and electromagnetic, the first two being characterized by an extremely small radius of action. At distances exceeding this radius, the interaction of nuclei and particles is determined by long-range electromagnetic forces arising between objects of the microworld having a special property - an electric charge. One manifestation of the action of such forces is the Coulomb barrier. What this phenomenon is and what physical processes it is associated with will be briefly described in this short article.
Nuclear fusion conditions
The concept of the Coulomb potential barrier is closely related to nuclear fusion reactions. These processes, during which the nuclei of light elements, starting with the simplest - hydrogen, - combine with great energy release, play a huge role in the Universe. It is nuclear fusion that makes it possible to form chemical elements heavier than hydrogen. Radiation from stars, including solar energy, also has this class of nuclear processes as its source.
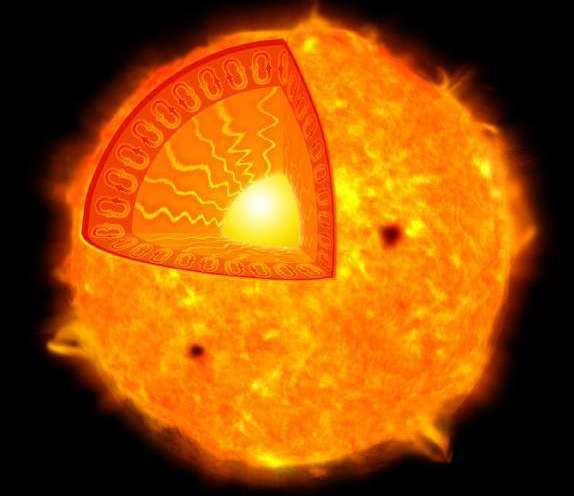
All reliably known synthesis reactions occur in high-temperature plasma - for this reason they are also called thermonuclear. The higher the temperature, the more efficient the reaction. In the central regions of the Sun, for example, the temperature exceeds 14 million degrees and the plasma is compressed to a density 150 times the density of water, but the Sun is a very inefficient thermonuclear reactor. The specific heat (per unit volume) of the human body significantly exceeds the solar, and a large total energy release of our luminary is achieved only due to its colossal mass and size.
The high temperature necessary for the synthesis of nuclei means their high kinetic energy - it is also needed to overcome the potential Coulomb barrier that nature posed to interacting nuclei.
Concept of a potential barrier
In order for the synthesis reaction to take place, two nuclei (for example, two protons - light hydrogen nuclei) must come closer to the distance of the strong interaction radius. It is very small - of the order of 10 -15 m. But in order to come so close to each other, they must overcome the increasing electrostatic (Coulomb) repulsion, reaching a maximum at the greatest approximation.
The region where the repulsive potential energy U, that is, the work that needs to be done to bring the particles closer to distance r, exceeds the kinetic energy of the incident particle, is called the potential Coulomb barrier. The formula for calculating this potential energy has the form U = Z 1 Z 2 e 2 / 4ΟΞ΅ 0 r, where Z 1 , Z 2 are the charge numbers of the nuclei, Ξ΅ 0 is the electric constant, and e is the elementary electric charge.
The smallest distance r min Μ΄ 10 -15 m, or 1 Fermi, is comparable with the size of the nuclei, and here lies the peak of the potential barrier U max . If a proton or other nucleus passes it with sufficient kinetic energy, it falls into the sphere of action of powerful nuclear forces and falls into the potential well created by them.
Quantum tunneling
A particle has the opportunity to overcome the Coulomb barrier, even if its energy does not reach U max . The simplest explanation for this quantum effect is based on the uncertainty relation for momentum p and x coordinate: ΞpΞx β₯ Δ§ / 2 (here Δ§ is the reduced Planck constant). It follows from it that the more limited a particle is by the value of one parameter, the more uncertain the value of another becomes. Such uncertainty of the momentum (associated with a direct relation to energy) or the coordinate allows the particle with some probability to be βon the other sideβ of the barrier.
As can be seen from the graph, the Coulomb barrier of the nucleus has an unequal width: it is large at the bottom, and decreases significantly closer to the top of the barrier. In other words, particles with low kinetic energy have little chance of tunneling through it. And the closer the particle energy is to the height of the barrier, the greater the probability of tunneling becomes.
Coulomb barrier height and nucleosynthesis
From the formula U = Z 1 Z 2 e 2 / 4ΟΞ΅ 0 r it can be seen that the height of the potential barrier directly depends on the charges of the interacting nuclei. A simple calculation can determine that for two protons the barrier height is about 1.1 MeV, which corresponds to a temperature of almost 13 billion degrees. It is clear why the Sun is so "inefficient" - because its central regions are colder by three orders of magnitude. However, synthesis is still going on there: approximately one hundred millionth part of the protons still has high enough energy to tunnel through the barrier. This turns out to be sufficient to provide the observed luminosity of the Sun.
For nuclei with a large charge, the barrier increases significantly. So, to combine carbon nuclei with the formation of magnesium, sodium or neon, an energy of 40 MeV or 450 billion degrees is already needed, but this reaction proceeds rather slowly even at a billion degrees in the centers of massive stars. So the existence of the Coulomb barrier and its magnitude, firstly, provide the Universe with a variety of chemical elements, and, secondly, give stars the opportunity to shine steadily for a long time.