This article talks about what quantization of energy is and what significance this phenomenon has for modern science. The history of the discovery of the discreteness of energy is presented, and the applications of atom quantization are demonstrated.
The end of physics

At the end of the nineteenth century, scientists faced a dilemma: at the then level of development of technology, all the possible laws of physics were discovered, described and studied. Students who had highly developed abilities in the field of natural sciences, teachers were not advised to choose physics. They believed that it was already impossible to become famous in it, only the routine work of studying small insignificant details remained. This was more suitable for an attentive person, rather than a gifted one. However, photography, which was more of an entertainment discovery, gave reason to think. It all started with simple inconsistencies. To begin with, it turned out that the light was not completely continuous: under certain conditions, burning hydrogen left a series of lines on the photographic plate instead of a single spot. It turned out further that helium spectra had more lines than hydrogen spectra. Then it turned out that the trail of some stars is different from others. And pure curiosity made researchers manually set one experiment after another in search of answers to questions. They did not think about the commercial application of their discoveries.
Plank and Quantum
Fortunately for us, this breakthrough in physics was accompanied by the development of mathematics. Because the explanation of what happened fit into incredibly complex formulas. In 1900, Max Planck, working on the theory of radiation from a completely black body, found out that energy quantization was taking place. Briefly about the meaning of this statement is quite simple. Any elementary particle can only be in some specific states. If you give a rough model, then the counter of such states can show the numbers 1, 3, 8, 13, 29, 138. And all other values between them are not available. The reasons for this we will reveal a little later. However, if you plunge into the history of this discovery, it is worth noting that the scientist himself until the end of his life considered the quantization of energy only a convenient mathematical trick, not endowed with a serious physical meaning.
Wave and mass
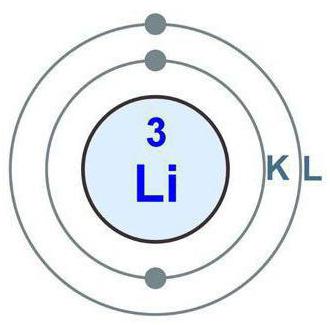
The beginning of the twentieth century was replete with discoveries related to the world of elementary particles. But the following paradox was a big mystery: in some cases, the particles behaved like objects with mass (and, accordingly, momentum), and in some - like a wave. After long and persistent disputes, I had to come to an incredible conclusion: electrons, protons and neutrons possess these properties simultaneously. This phenomenon was called wave-particle duality (in the speech of Russian scientists two hundred years ago, a particle was called a particle). Thus, the electron is a certain mass, as if smeared into a wave of a certain frequency. An electron that revolves around the nucleus of an atom infinitely superimposes its waves on top of each other. Consequently, only at certain distances from the center (which depend on the wavelength) the electron waves, while rotating, do not cancel each other out. This occurs when, when the “head” of the wave electron is superimposed on its “tail”, the maxima coincide with the maxima and the minima coincide with the minima. This explains the quantization of atomic energy, that is, the presence in it of strictly defined orbits on which an electron can exist.
Spherical nanocon in vacuum

However, real systems are incredibly complex. Obeying the logic described above, we can still understand the system of electron orbits in hydrogen and helium. However, rather complicated calculations are already required. To learn how to understand them, modern students study the quantization of particle energy in a potential well. To begin with, they choose the perfect shape of the pit and one single model electron. For them, they solve the Schrödinger equation, find the energy levels at which the electron can be. After they learn to look for dependencies, introducing more and more variables: the width and depth of the well, the energy and frequency of the electron lose certainty, adding complexity to the equations. Next, the shape of the pit changes (for example, it becomes square or jagged in the profile, its edges lose symmetry), hypothetical elementary particles with given characteristics are taken. And only then learn to solve problems in which the quantization of the radiation energy of real atoms and even more complex systems appears.
Impulse, angular momentum
However, the energy level of, say, an electron is an even more or less understandable quantity. All, one way or another, but represent that a higher energy of central heating batteries corresponds to a higher temperature in the apartment. Accordingly, quantization of energy can still be imagined speculatively. There are also such concepts in physics that it is intuitively difficult to comprehend. In the macrocosm, momentum is the product of speed and mass (do not forget that speed, like momentum, are vector quantities, that is, they depend on the direction). It is thanks to the impulse that it is clear that a slowly flying medium-sized stone will only leave a bruise if it hits a person, while a small bullet released at great speed will pierce the body through and through. In the microcosm, the momentum is such a quantity that characterizes the connection of a particle with the surrounding space, as well as its ability to move and interact with other particles. The latter directly depends on energy. Thus, it becomes clear that the quantization of energy and momentum of a particle must be interconnected. Moreover, the constant h, which denotes the smallest possible portion of a physical phenomenon and shows the discreteness of values, is included in the formula of both energy and momentum of particles in the nanoworld. But there is a concept even farther from intuitive awareness - the moment of impulse. It refers to rotating bodies and indicates what mass and with what angular velocity rotates. Recall that the angular velocity shows the amount of rotation per unit time. The angular momentum is also able to communicate the distribution of matter of a rotating body: objects with the same mass but concentrated near the axis of rotation or at the periphery will have different angular momentum. As the reader probably already guesses, in the world of the atom there is a quantization of the energy of the angular momentum.
Quantum and laser

The effect of the discovery of discreteness of energy and other quantities is obvious. A detailed study of the world is possible only thanks to a quantum. Modern methods of studying matter, the use of various materials, and even the science of creating them, are a natural continuation of understanding what quantization of energy is. The principle of operation and the use of a laser is no exception. In general, a laser consists of three main elements: a working fluid, a pump, and a reflector mirror. The working fluid is chosen so that there are two relatively close levels for electrons. The most important criterion for these levels is the electron lifetime on them. That is, how much the electron is able to hold out in a certain state before moving to a lower and more stable position. Of the two levels, the upper should be the more long-lived. Then pumping (often an ordinary lamp, sometimes an infrared lamp) gives the electrons enough energy so that they all gather at the upper energy level and accumulate there. This is called inverse level population. Next, a single electron goes into a lower and more stable state with the emission of a photon, which causes a breakdown of all electrons down. A feature of this process is that all the resulting photons have the same wavelength and are coherent. However, the working fluid, as a rule, is quite large, and flows are generated in it, directed in different directions. The role of the reflector is to filter out only those photon streams that are directed in one direction. As a result, the output is a narrow intense beam of coherent waves of the same wavelength. At first, this was considered possible only in a solid. The first laser had an artificial ruby as a working fluid. Now there are lasers of all kinds and types - on liquids, gas, and even chemical reactions. As the reader sees, the main role in this process is played by the absorption and emission of light by an atom. The quantization of energy in this case is only the basis for the description of the theory.
Light and electron
Recall that the transition of an electron in an atom from one orbit to another is accompanied by either emission or absorption of energy. This energy appears in the form of a quantum of light or a photon. Formally, a photon is a particle, but it differs from other inhabitants of the nanoworld. A photon has no mass, but it has momentum. This was proved by the Russian scientist Lebedev in 1899, clearly demonstrating the pressure of light. A photon exists only in motion and its speed is equal to the speed of light. This is the fastest object possible in our universe. The speed of light (standardly denoted by a small Latin "s") is about three hundred thousand kilometers per second. For example, the size of our galaxy (not the largest by cosmic standards) is about one hundred thousand light years. Faced with matter, the photon gives it its energy completely, as if dissolving in this case. The energy of a photon that is released or absorbed during the transition of an electron from one orbit to another depends on the distance between the orbits. If it is small, infrared radiation with low energy is released; if large, ultraviolet is obtained.
X-ray and gamma radiation
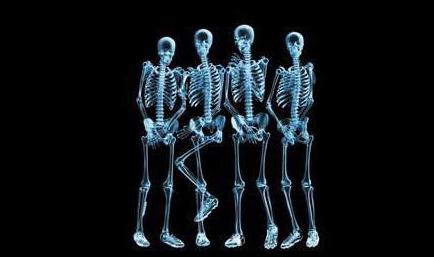
The electromagnetic scale after ultraviolet radiation contains x-rays and gamma radiation. In general, they overlap in a fairly wide range in terms of wavelength, frequency and energy. That is, there is an x-ray photon with a wavelength of 5 picometers and a gamma photon with the same wavelength. They differ only in the method of receipt. X-rays occur in the presence of very fast electrons, and gamma radiation is obtained only in the decay and fusion of atomic nuclei. An x-ray is divided into soft (with its help the human lungs and bones are translucent) and hard (usually needed only for industrial or research purposes). If you accelerate the electron very much and then brake it sharply (for example, by directing it into a solid), then it will emit x-ray photons. When such electrons collide with matter, electrons from the lower shells escape from the atoms of the target. In this case, the electrons of the upper shells take their place, during the transition also emitting x-rays.
Gamma rays occur in other cases. The nuclei of atoms, although they consist of many elementary particles, are also small in size, which means that they are characterized by quantization of energy. The transition of nuclei from an excited state to a lower one is precisely accompanied by the emission of gamma rays. Any reaction of decay or fusion of nuclei proceeds, including with the appearance of gamma-photons.
Nuclear reaction
We mentioned a little above that atomic nuclei also obey the laws of the quantum world. But in nature there are substances with such large nuclei that they become unstable. They tend to break up into smaller and more stable components. They, as the reader probably already guesses, include, for example, plutonium and uranium. When our planet formed from a protoplanetary disk, there was a certain amount of radioactive substances in it. Over time, they decayed, turning into other chemical elements. But nevertheless, a certain amount of undecayed uranium has reached our days, and by its quantity one can judge, for example, the age of the Earth. For chemical elements that have natural radioactivity, there is such a characteristic as half-life. This is the period of time during which the number of remaining atoms of this species will be halved. The half-life of plutonium, for example, occurs in twenty-four thousand years. However, in addition to natural radioactivity, there is also a forced one. If atomic nuclei are bombarded with heavy alpha particles or light neutrons, they crack. In this case, three types of ionizing radiation are distinguished: alpha particles, beta particles, gamma rays. Beta decay leads to a change in the charge of the nucleus per unit. Alpha particles take two positrons from the nucleus. Gamma radiation has no charge and does not deviate from the electromagnetic field, but has the highest penetrating power. Quantization of energy occurs in all cases of decay of the nucleus.
War and Peace
Lasers, X-rays, the study of solids and stars are all peaceful applications of quantum knowledge. However, our world is full of threats, and everyone seeks to protect themselves. Science serves military purposes too. Even such a purely theoretical phenomenon as quantization of energy has been put on guard of the world. Determining the discreteness of any radiation, for example, formed the basis of nuclear weapons. Of course, there are only a few of its combat applications - the reader probably remembers Hiroshima and Nagasaki. All other reasons to press the treasured red button were more or less peaceful. Also, the issue of radioactive pollution of the environment always remains. For example, the half-life of plutonium indicated above makes the terrain into which this element enters unsuitable for use for a very long time, practically for the geological era.
Water and wires
Let's return to the peaceful use of nuclear reactions. This, of course, is about generating electricity through fission of nuclei. This process looks like this:
First, free neutrons appear in the reactor core, and then they hit a radioactive element (usually an uranium isotope), which undergoes alpha or beta decay.
So that this reaction does not go into an uncontrolled stage, the reactor core contains so-called moderators. As a rule, these are rods made of graphite, which absorb neutrons very well. By adjusting their length, you can monitor the reaction rate.
As a result, one element turns into another, and an incredible amount of energy is released. This energy is absorbed by a container filled with so-called heavy water (instead of hydrogen in deuterium molecules). As a result of contact with the reactor core, this water is heavily polluted by radioactive decay products . It is the disposal of this water that is the biggest problem of nuclear power at the moment.
The second is placed in the first water circuit, the third in the second. The water of the third circuit is already safe to use, and it is she who spins the turbine, which produces electricity.
Despite such a large number of intermediaries between the directly emitting cores and the end user (let's not forget about the tens of kilometers of wires on which power loss also occurs), this reaction gives incredible power. For example, a single nuclear power plant can supply electricity to an entire area with many industrial plants.